Alfred Wegener’s Pioneering Continental Drift Theory
In the annals of geophysics, a significant turning point arrived in 1912 when Alfred Wegener, a German geophysicist, introduced the Continental Drift Theory (CDT). Wegener’s theory reshaped our understanding of the Earth’s history and geological transformations.
Wegener’s ground-breaking theory posited the existence of a colossal supercontinent named Pangaea, a term derived from Greek and meaning “all lands.” This supercontinent dominated the ancient world, enveloped by a vast ocean called Panthalassa.
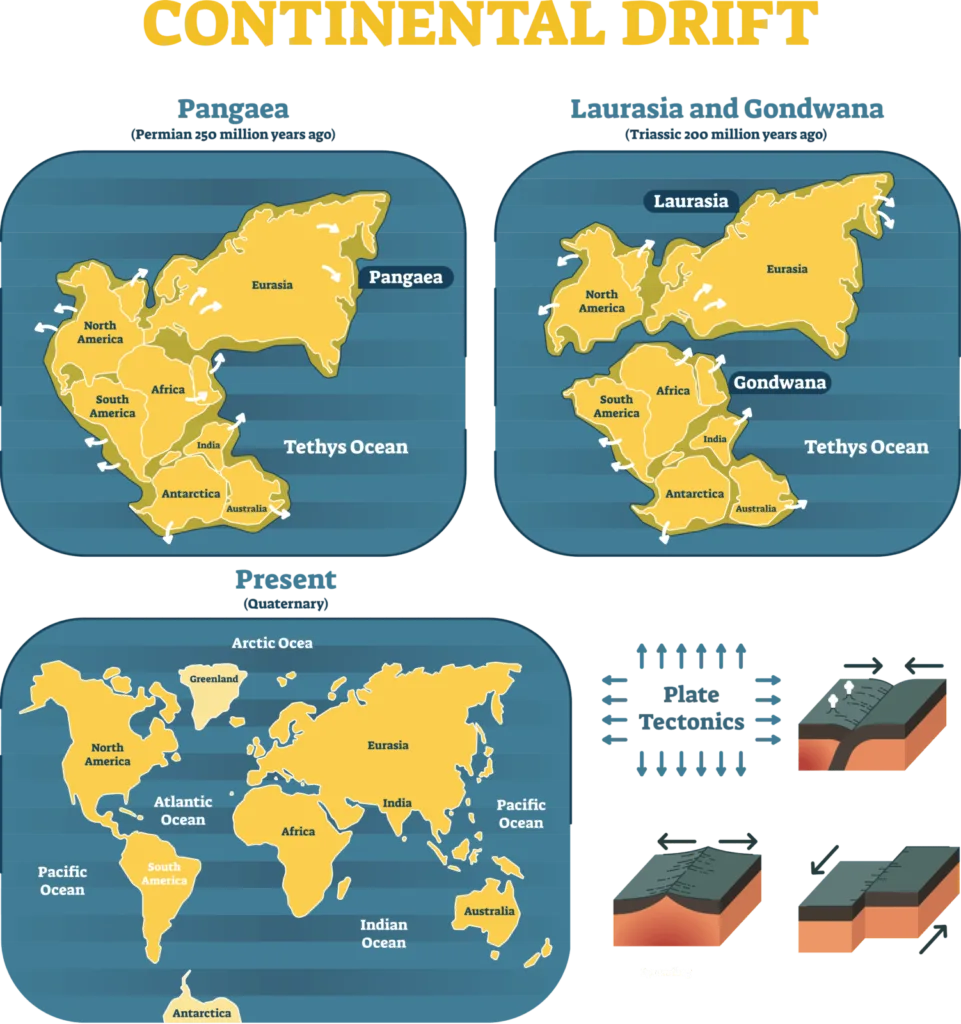
In Wegener’s vision, Pangaea was not a monolithic mass but was separated by a substantial body of water known as the Tethys Sea. This aquatic divider cleaved Pangaea into two immense landmasses: Laurentia, alternatively called Laurasia, occupied the northern realms, while Gondwanaland held sway in the southern domains, positioned below the Tethys Sea.
The grand narrative of continental drift set in motion approximately 200 million years ago. To be more precise, this geological journey commenced during the Mesozoic Era, within the Triassic Period and, in a more refined timeframe, the Late Triassic Epoch. At this point in the Earth’s chronicle, the continents underwent a profound transformation. They began to fracture, separating from their once-conjoined counterparts and embarking on distinctive trajectories through geological epochs. Alfred Wegener’s Continental Drift Theory stands as a landmark in our comprehension of Earth’s evolution, reshaping how we perceive the movement and history of continents.
Forces Underlying Continental Drift, as Proposed by Wegener
Alfred Wegener’s Continental Drift Theory not only proposed the existence of a grand supercontinent and the separation of landmasses but also delved into the forces propelling these transformative movements. According to Wegener, this geological journey was influenced by several significant forces acting upon Earth’s crust. These forces are instrumental in driving the continents in two key directions.
Equatorward Drift: One of the forces Wegener identified was the drift towards the equator. This equatorward movement resulted from a complex interplay of forces, including the influence of gravity. Additionally, it was attributed to the pole-fleeing force, a centrifugal effect arising from the Earth’s rotation. In a similar vein to the buoyancy principle, which allows ships to float on water, these forces played a role in propelling continents toward the equator.
Westward Drift: The second directional influence Wegener postulated was the westward drift. He attributed this movement to tidal currents generated by Earth’s rotation. Since the Earth rotates from west to east, the tidal currents act in the opposite direction, from east to west, according to Wegener’s framework.
The role of centrifugal force was an integral aspect of Wegener’s explanation. This force increases as one moves from the poles toward the equator, leading to the phenomenon known as “pole-fleeing.”
Wegener contended that the combined impact of these forces, operating over vast periods, was the driving mechanism behind the gradual drifting of continents. It was a geological saga that extended across millions of years and, in Wegener’s view, is still in motion, reshaping the face of our planet.
Supporting Evidence for Continental Drift Theory
Alfred Wegener’s Continental Drift Theory was met with skepticism when it was first introduced, but it brought forth a range of intriguing evidence to bolster its claims. One key piece of evidence was the apparent affinity of physical features on continents that appeared to align compellingly.
- Continental Contours: One striking observation was the remarkable similarity in the shapes of coastlines and landmasses on opposite ocean sides. For example, the bulge of Brazil on the South American coast seemed to exhibit a snug fit with the Gulf of Guinea in Africa. Greenland appeared to have a fitting counterpart in the Ellesmere and Baffin Islands of Canada. Alongside this, the west coasts of India, Madagascar, and Africa suggested that they might have once been connected.
- Mid-Atlantic Ridge Alignment: Another piece of this geological puzzle was the alignment of land features along the mid-Atlantic ridge. The continents of North and South America, on one side, and Africa and Europe, on the other, seemed to interlock like pieces of a grand geological jigsaw puzzle.
- Ancient Mountain Chains: very old mountain chains further corroborated the idea of continental drift. The Caledonian and Hercynian mountains of Europe and the Appalachian Mountains in the USA displayed geological similarities, hinting at a shared history.
However, Wegener’s theory faced criticism. Detractors pointed out that coastlines were not static but subject to change over time, and the geological affinity between mountains was not always apparent. They also argued that alternative explanations for the observed similarities could be proposed.
Despite these criticisms, Wegener’s Continental Drift Theory laid the groundwork for the revolutionary concept of plate tectonics, which is now widely accepted as the driving force behind the movement of Earth’s continents.
Botanical and Fossil Evidence for Continental Drift
Alfred Wegener’s Continental Drift Theory was supported by various evidence, particularly botanical and fossil findings. This evidence helped illustrate the connection between continents and the theory that they were once part of a larger landmass.
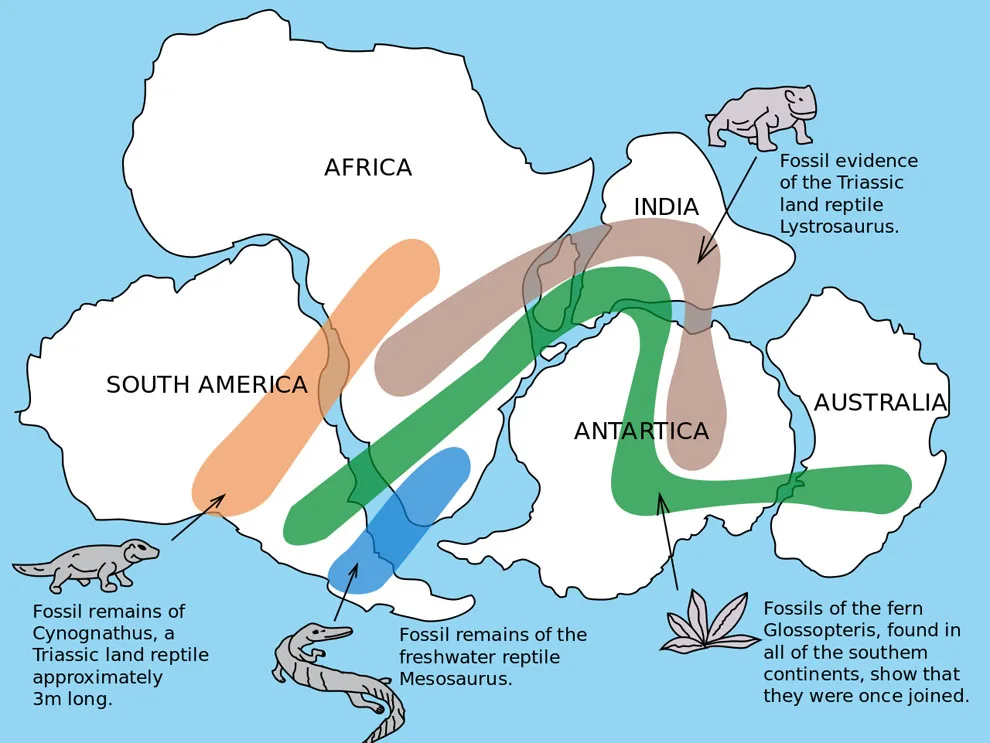
- Glossopteris Vegetation: Glossopteris vegetation in Carboniferous rocks across India, Australia, South Africa, the Falkland Islands, and Antarctica suggests a shared botanical history. These regions were believed to be part of the ancient supercontinent Gondwana, which began to break apart. However, criticism arose as similar vegetation was found in seemingly unrelated regions like Afghanistan, Iran, and Siberia.
- Fossil Distribution: Observations of Lemurs in India, Madagascar, and Africa led to the hypothesis of a landmass called “Lemuria” that once connected these areas. Mesosaurus fossils, adapted to shallow brackish water, were found exclusively in South Africa and Brazil. However, these regions are now separated by a vast ocean, which raises questions about their shared history. Similar fossils were also discovered in unrelated parts of the world, casting doubt on this evidence.
- Polar Wandering: The theory of polar wandering, where the position of the poles shifted over time due to plate tectonics, supported the concept of continents in motion. Critics, however, argued that the poles may have shifted without continents moving.
- Rocks of the Same Age: Ancient rocks, around 2 billion years old, along Brazil’s coast seemed to match those found in western Africa. Nevertheless, rocks of similar age and characteristics were discovered in other regions globally.
- Tillite Deposits: Sedimentary rocks known as tillite, formed from glacier deposits, were found in the Gondwana regions of India, Africa, Falkland Islands, Madagascar, Antarctica, and Australia. These shared geological features implied a common history among these land masses.
- Placer Deposits: Valuable placer deposits of gold on the Ghana coast were traced back to the source in Brazil, supporting the idea that these continents were once adjacent. These deposits suggest that gold from the Brazil plateau contributed to Ghana’s mineral wealth.
Drawbacks of Continental Drift Theory
Although Wegener’s theory was groundbreaking, it had several limitations:
- It did not explain why the drift commenced during the Mesozoic era and not earlier.
- The theory overlooked the role of oceans.
- Many of the proofs relied on general assumptions.
- Wegener’s proposed factors for drift, such as Earth’s gravity, the buoyancy of the seas, and tidal currents, were considered insufficient to cause such massive movements.
Modern theories like Plate Tectonics offer a different perspective, acknowledging the existence of Pangaea and related landmasses while providing alternative explanations for the causes of continental drift. Despite its shortcomings, Wegener’s theory marked a significant milestone in the study of tectonics and laid the foundation for future theories like seafloor spreading and plate tectonics.
Seafloor Spreading and Convection Current Theory
In 1960, American geologist Harry Hess introduced the concept of Seafloor Spreading, a critical component in understanding continental drift and the broader theory of plate tectonics. Seafloor spreading sheds light on the mechanisms behind the movement of Earth’s lithospheric plates.
When oceanic plates separate, tensional stress fractures the lithosphere. From these fractures, molten basaltic magma rises and solidifies on the ocean floor, forming new seafloor. Over time, the newly created seafloor, known as oceanic crust, moves away from the mid-ocean ridge. It is subsequently replaced by even newer seafloor, initiating a continuous cycle. As a result, older rocks are found farther away from the spreading zone, while younger rocks are closer to it.
To fully grasp the concept of seafloor spreading, one must delve into the Convection Current Theory (CCT) and the role of paleomagnetism.
Convection Current Theory (CCT)
In the 1930s, British geologist Arthur Holmes laid the foundation for the Convection Current Theory (CCT). According to CCT, convection currents within the mantle arise from temperature variations generated by radioactive elements.
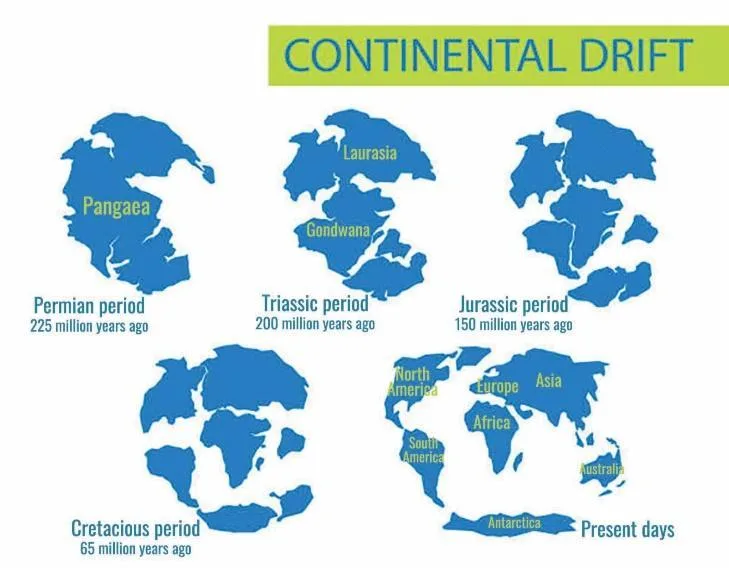
These convection currents are the primary driving force responsible for the movement of Earth’s lithospheric plates, an integral aspect of seafloor spreading and plate tectonics. When the descending limbs of these convection currents exert a negative pressure on the lithosphere, they cause tectonic plates to converge. Along convergent boundaries, typical landforms include trenches, volcanic arcs, and fold mountains.
Conversely, the ascending limbs of the convection currents create a positive pressure on the lithosphere, leading to the divergence of tectonic plates. This process gives rise to oceanic ridges in seas, rift valleys, and rift lakes on land.
While heat from Earth’s interior is transferred to the surface through phenomena such as volcanoes, springs, and geysers, this heat is relatively minor compared to solar heat. However, deep ocean ecosystems rely on the heat generated at the ocean floor from the Earth’s interior. Bacteria near volcanic vents use a process known as chemosynthesis, which involves harnessing chemicals in vent fluid to produce energy. This adaptation enables their survival in light-deprived ocean depths, where photosynthesis is unattainable, as sunlight cannot penetrate these depths.
Paleomagnetism: A Window into Earth’s History
Paleomagnetism played a pivotal role in resurrecting the continental drift hypothesis and transforming it into the theories of Seafloor Spreading and Plate Tectonics.
In essence, paleomagnetism involves scrutinizing Earth’s magnetic field’s historical record through the magnetic fields embedded in rocks, sediments, and archaeological materials. This approach has unearthed remarkable insights into our planet’s magnetic history.
The cornerstone of this study lies in rocks, particularly those formed through underwater volcanic activity, primarily composed of basalt—a type of rock rich in iron and low in silica. Crucially, basalt harbors magnetic minerals. During the solidification process of basalt, these magnetic minerals align themselves in response to the prevailing magnetic field. As they lock into position, they effectively capture a historical snapshot of the magnetic field’s orientation at the time.
Through paleomagnetic investigations of rocks, scientists have unveiled a fascinating revelation: Earth’s magnetic field’s orientation has alternated with significant frequency throughout geological epochs. This phenomenon is termed “geomagnetic reversal,” wherein the polarity of Earth’s magnetic field undergoes a complete flip. By studying rocks of various ages, researchers can decipher the magnetic history of our planet, shedding light on the enigmatic process of magnetic field reversals. This magnetic journey into Earth’s past has been instrumental in validating and expanding our understanding of continental drift, Seafloor Spreading, and the broader framework of Plate Tectonics.
Paleomagnetism: A Strong Pillar of Evidence for Seafloor Spreading and Plate Tectonics
Paleomagnetism, the study of Earth’s ancient magnetic field imprinted in rocks, unveils compelling evidence supporting the concepts of Seafloor Spreading and Plate Tectonics, particularly along the mid-ocean ridges where the oceanic crust is continuously expanding.
By closely examining paleomagnetic rocks on both sides of these underwater mountain ranges, a striking pattern emerges alternating magnetic rock stripes. One stripe exhibits normal magnetic polarity, while the next displays reversed polarity.
This magnetic striping phenomenon is a linchpin in the case of Seafloor Spreading. As tectonic plates drift apart at these ridges, magma rises from the depths, solidifies into elongated bands of rock on either side of the vent, and acquires the prevailing magnetic field’s polarity at the time. Subsequently, these rock bands migrate away from the ridge as new ones form in the wake of magnetic field reversals. This process recurs over time, resulting in the distinct alternating patterns of magnetic striping on the seafloor.
Several key pieces of evidence support this idea:
1. Nature of Oceanic Rocks Around Mid-Ocean Ridges:
- Rocks on both sides of the oceanic ridges at equidistant locations from the ridge crest share similarities in composition, age, and magnetic orientation.
- The rocks closest to the mid-ocean ridges exhibit normal polarity, denoting their youth, while the age of rocks increases with distance from the ridge.
- Oceanic crust rocks near the ridges are significantly younger than those of the continental crust.
2. Distribution of Earthquakes and Volcanoes Along Mid-Ocean Ridges:
- An elevated temperature gradient near the ridges, indicating an upwelling of molten material from the mantle, serves as a telltale sign.
- Earthquake foci in ridge areas are shallow, whereas regions like the Alpine-Himalayan belt and the Pacific’s rim witness deep-seated earthquakes, a consequence of subduction.
This compilation of geological and geophysical evidence bolsters the compelling case for Seafloor Spreading and its integral role in Plate Tectonics.
For Plate Tectonic Theory VISIT HERE
Mains Question
Q. What do you understand by the theory of continental drift? Discuss the prominent evidence in its support. (UPSC Mains 2013)